The EV sector world-over is witness to significant growth with batteries having an integral part of the sector. Recently, we see major demand for EVs around the world, and a majority of them are powered by Lithium-ion batteries. These batteries have the potential to offer many benefits. The safety of lithium-ion batteries is of utmost importance in EVs and thus the basic understanding of the various battery hazards and design challenges is critical for all key stakeholders like battery manufacturers, OEMs, engineers, government, regulators, etc.
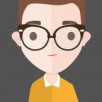
Lorem ipsum dolor sit amet, nec in adipiscing purus luctus, urna pellentesque fringilla vel, non sed arcu integer, mauris ullamcorper ante ut non torquent.
Understanding the fundamentals…
The lithium-ion battery was made possible by the discovery of lithium cobalt oxide (LiCoO2), which allows the extraction of lithium ions and the creation of large amounts of vacancies (without a crystal change) up to the removal of half of the existing ions. The pairing of LiCoO2 with graphite allows the intercalation of lithium ions between the graphene layers that occupy the interstitial site
The lithium ions travel during charge from the positive electrode (the cathode) through a solid or liquid electrolyte to the negative electrode (the anode) and, during discharge, in the opposite direction. At each electrode, the ion either maintains its charge and intercalates into the crystal structure occupying interstitial sites in existing crystals on the anode side, or reoccupies a vacant site in the cathode that formed when the lithium-ion left that crystal. While transferring the ion, the host matrix gets reduced or oxidized, which releases or captures an electron.
The search for new cathode materials is driven in part by important disadvantages of LiCoO2. The battery has a core temperature of 40–70°C and may be susceptible to some low-temperature reactions. But at 105–135°C it is very reactive and an excellent oxygen source for a safety hazard called a thermal runaway reaction, in which highly exothermic reactions create temperature spikes and accelerate rapidly with the release of extra heat (Roth 2000).
Replacement materials for LiCoO2 are less prone to that failure. The compounds replace parts of the cobalt with nickel and manganese to form Li(NixMnyCoz)O2 compounds (with x + y + z = 1), often referred to as NMC as they contain nickel, manganese, and cobalt; or they exhibit a completely new structure in the form of phosphates (e.g., LiFePO4) (Daniel et al. 2014). These cathode materials all exhibit capacities in the range of 120–160 Ah/kg at 3.5–3.7 V, resulting in a maximum energy density of up to 600 Wh/kg.
When packaged in real devices, however, much inactive material mass is added and the energy density tends to drop to 100 Wh/kg on the pack level. To push for higher energy density, researchers have sought higher capacity and higher voltage—and found them in lithium- and manganese-rich transition metal oxides. These compounds are essentially the same materials as NMC but an excess of lithium and higher amounts of manganese replace nickel and cobalt. The higher amounts of lithium (as much as 20 percent more) allow the compounds to have higher capacity (Thackeray et al. 2007) and a higher voltage, resulting in cathodes with up to 280 Ah/kg when charged up to 4.8 V. However, these new compounds show stability problems and tend to fade fast.
Cost as a concern
Further cost reduction is possible through the optimization of manufacturing schemes. Lithium-ion batteries are manufactured in sets of electrodes and then assembled in cells. The active material is mixed with polymer binders, conductive additives, and solvents to form a slurry that is then coated on a current collector foil and dried to remove the solvent and create a porous electrode coating. The solvent of choice, N-methylpyrrolidone (NMP), is considered an indirect material (it is needed for production but not contained in the final device), but it is expensive, exhibits flammable vapors, and is highly toxic. The flammable vapors of NMP require all processing equipment during the production of electrodes to be explosion-proof, meaning all spark-producing electrical components need to be shielded from the vapors and spaces need to be highly ventilated to keep vapor concentrations low. These measures increase the capital cost of such equipment considerably.
In addition, the electrode manufacturing plant is required to recapture the solvent from its exhaust stream, distil it, and recycle it. This is again an additional cost. Further cost reductions will be achieved through greater knowledge of transport mechanisms and electrode architecture implications for electrochemical performance. Current research is largely focused on modelling and simulation to understand molecular mechanisms and improve the design of electrodes, electrode stacks, and battery cells. Thicker electrodes and a tremendous reduction in inactive materials will improve energy density at lower cost, reduce direct costs, and possibly enable much shorter and less energy intensive battery formation cycling.
In the case of high voltage batteries, there are standard protocols as the high-speed EV manufacturers who use these high voltage batteries have to get proper certification from the govt. But in the case of low voltage batteries which are used by low-speed EVs, there aren’t mandatory certifications. Hence, standard protocols are not followed. Standard protocols include the type of connectors, wires to be used, and also battery testing standards.
CKD Models
Speaking of India, the entire EV market of India is based on the CKD model in which the entire EV is imported by the OEM into segments and assembled in India. OEM does it to reduce the import duty. Secondly, to showcase that they have various options available for the customers, they import EVs with different design options on the same voltage platform. This means even though the voltage is the same every other EV will require a different battery design because the chassis design will be different.
Sheet Metal Challenges in Battery Designing
The thickness and strength of the sheet metal are very important. A slight change in the thickness of the sheet metal can cause a lot of problems. If the thickness is increased, then the overall load of the EV will increase. That will further increase the rate of discharge of the battery.
If the thickness is less, then, in the long run, the chances of the battery box getting destroyed cannot be neglected. The weight of the cells will impact the battery box if its thickness is less than what is required.
Lack of Skilled Engineers
As of now Lithium Battery Manufacturing in India is very new. So we don’t have engineers with even the basic skills required to design lithium batteries. It’s mostly because of the gap between education and industry. Now regarding the skilled engineers and workforce, the industry experts need to develop some standards and protocols, and share the same with the education department of the govt. so that new education policies can be developed. This can help to fulfil the skill gap in the industry.
Logistical Challenges
The logistic challenges can be understood in the below given 3 points.
First, and perhaps most evident to many of us, was the COVID pandemic’s unprecedented strains on global supply networks, as well as the accompanying sequence of lockdowns and restrictions that varied in timing and intensity from nation to country.
Second, the Suez Canal blockade last year impacted 12% of world commerce since the canal transports nearly one million barrels of oil and 8% of liquefied natural gas every day.
Third, the impact of logistics and supply chain operations on the environment is becoming more recognized. Governments must establish more sustainable supply chain practices if they are to reach their emissions objectives and pledges. The COP26 meeting in Glasgow in November placed a major emphasis on transportation, especially freight and logistics. If a sustainable future is to be realized, business as usual is no longer an option.
Steps by the Govt of India
1. India has introduced the PLI scheme to set up a domestic lithium-ion cell manufacturing industry.
2. India has introduced an Rs. 76000 crore PLI scheme to set up the domestic semiconductor manufacturing industry.
3. India is working towards setting up an industry for manufacturing EVs within the country.
4. All these things will for sure lower our dependency on Import.
These steps will lower the dependency on imports hence increasing the efficiency and use of Lithium-Ion batteries within the country and enhancing their application.
Your Comment Please